Inspired by the Nobel Prize Experiments for High School Students program, we also give first-year physicist-engineer students the opportunity to experiment on topics related to both Nobel Prizes and faculty research topics.
Measurement sessions in 2024 (click for details):
For those who took part in the secondary school program, we will try - to a limited extent - to organize other measurements instead of the ones taken there.
Requirements:
The basic requirement is the attendance of the sessions, and the preparation of a lab report about your favorite session (free choice). The length of the report should be around 15000-20000 characters with spaces, including figure captions, + around 3-5 figures. You have free choice for the style (word, latex, etc). The lab reports will not undergo a rigorous review, but they should reflect your interest, also demonstrating what you have learned from the measurement. Careless and negligent reports, however, are not welcome. The reports should be prepared individually (not in pairs).
Attendance: as we told, this course requires a lot of work from us serving your benefit, so please attend all the sessions unless you have a very serious reason for the absence. A single absence can be replaced by an extra, duly written lab report. One more additional absence is only possible in rare and well-justified cases, relying on the consultation with the class responsible (András Halbritter) in advance.
Short descriptions of the sessions:

Superconductivity
Dutch physicist Heike Kamerlingh Onnes was the first to carry out experiments at temperatures very close to absolute zero after building his helium liquefier apparatus in 1911. Within a few months, his low-temperature measurements led to a very astonishing discovery: he found that the resistance of a mercury filament drops to zero below 4.19 K. Later it turned out that many materials (e.g. lead, tin, aluminium) behave as perfect conductors, so-called superconductors, at sufficiently low temperatures. Kammerling Onnes' work was awarded the Nobel Prize just two years later, in 1913. Since this early discovery, superconductivity has been a constant focus of modern physics. If we look only at the most recent achievements, Nobel Prizes were awarded in 1972, '73, '87 and 2003 for theoretical or experimental work on superconductivity. Of these, the Nobel Prize for high-temperature superconductors (1987) is of particular technical importance, as it made it possible to achieve superconductivity in liquid nitrogen, which is considerably cheaper than liquid helium.
Superconductivity is used in many areas of life, from magnetically levitated trains to superconducting magnets in medical MRI machines. Superconducting circuits have long been one of the most sensitive magnetic field sensors (SQUIDs), and today superconducting nanostructures are proving to be the most suitable for building quantum computers.
During the measurement, you can experiment with high-temperature superconductors, measure the resistance of a superconducting wire going to zero, and test a superconducting ring with an undamped current circulating without an external voltage source. To measure the magnetic field, you can use another Nobel Prize-winning discovery (2007), the so-called giant magnetoresistance sensor. We will measure in liquid nitrogen (at around -200 degrees Celsius), giving you an insight into the world of low temperature measurements and a chance to try out how to automatically collect data with modern instruments and computer-controlled measurements.
You can find a detailed description of the measurement here.

Atomic Nanowires
Even the ancient Greeks assumed that matter was made up of atoms. This hypothesis was proven by a series of experiments in the early 20th century. However, it was not until 1981 that we were able to image atoms on the surface of matter. That was when Gerd Binnig and Heinrich Rohrer built the first scanning tunnelling microscope, for which they were awarded the Nobel Prize five years later.
The operation of the scanning tunnelling microscope (STM) is based on wave nature of electrons, which means that a current flows between two pieces of metal brought close together even if they do not touch. This current is called the quantum mechanical tunnelling current, which has the interesting property that it depends very sensitively on the distance between the two metals: if the gap width is reduced by just half an atom-atom distance, the current increases tenfold. If a metal needle cut with scissors is brought close to a metal surface, a significant part of the tunnel current flows through the single atom closest to the surface. During the measurement, the needle is scanned parallel to the sample surface, while a control circuit is used to move the needle in a direction perpendicular to the surface so that the measured tunnel current is always constant, i.e. the needle moves at a nearly constant distance from the sample surface. By recording the movement of the needle on a computer, an image of the surface can be taken at atomic resolution.
During the measurement, you will be shown how to build an STM, and then you will be able to experiment with a setup similar to an STM, which, although cannot scan in three dimensions, but will allow you to measure the conductivity of the thinnest nanowire, with current flowing through a single atom.
You can find a detailed description of the measurement here.


Holography
In 1947, the Hungarian-born electrical engineer and physicist Dénes Gábor (1900-1979) conceived the basic idea of holography: light from an object does not pass through a lens that would image it onto a photosensitive film (as in conventional photography), but is directly projected onto the photosensitive plate, together with another, so-called reference light wave. When the light wave from the object and the reference light wave are added together, an interference image is formed on the photosensitive plate, which records the full three-dimensional information about the wave from the object. To obtain a hologram image, a coherent light source emitting monochromatic light is required (e.g. sunlight or a traditional light bulb is not suitable). It is no wonder that the field of holography only made spectacular progress in the early 1960s with the invention of the lasers. However, the few years that followed led to the development of flourishing fields of research such as holographic optics, holographic interferometry, computer holography and reflection holography. This was enough to convince the Swedish Academy of Sciences committee to award the Nobel Prize in Physics to Dénes Gábor in 1971. Since then, holography has expanded into many other important areas, such as holographic data storage and holographic security.
In addition to reproducing a spectacular three-dimensional image, a particularly exciting feature of a hologram is that information is distributed differently from a traditional information storage media (such as a photograph, DVD or magnetic hard disk drive). Even when a hologram is broken into small pieces, the complete information of the object is preserved in each piece.
You will learn the tricks of holography, and everyone can make a hologram for themselves.
You can find a detailed description of the measurement here.
From Photoelectric Effect to Quantum Communication
A visit to the Optics Laboratory of the Department of Atomic Physics will give you the opportunity to learn about experiments and measurement methods that use light to probe the mysteries of the structure of matter, understand the operation of some of the most advanced light sources and finally gain insights into quantum optics methods of quantum communication.
The deeper understanding of the structure of matter, the exploration of the physics of the microworld, began just over a century ago with the application of radically new methods. While the laws of classical physics can be understood by direct experience, the structure of atoms or the rules of quantum mechanics can only be understood by very indirect observations and measurements. Light is of great help in indirectly exploring the microworld. Bohr's model of the atom (Nobel Prize: 1922) was made successful by his ability to explain the lines in the spectrum of light emitted by a hydrogen atom. During the lab visit, we will measure the spectral lines of hydrogen gas. The spectral line analysis led to the discovery of many new elements, such as the discovery of noble gases by Rayleigh (Nobel Prize: 1904). Einstein's (Nobel Prize: 1921) theoretical work was needed to understand the so-called photoelectric phenomenon.
During the lab visit, we will also measure the temperature radiation spectrum. We will also examine some special light sources and, using a mercury vapor lamp, repeat one of the fundamental experiments in quantum physics, the study of the photoelectric phenomenon. We will understand the conditions needed to produce laser light (Basov, Prokhorov, Townes Nobel Prize: 1964) and the properties of laser light. We also find out why the development of the blue light-emitting diode was so important that Japanese researchers Akasaki, Amano and Nakamura were awarded the Nobel Prize in 2014. Finally, we look at the mysteries of quantum optics (Nobel Prizes 2005 and 2012) and quantum communications (Nobel Prize 2022).
You can find the detailed description of the measurement here.

Control a nuclear reactor!
In 1932, James Chadwick discovered the neutron, for which he was awarded the Nobel Prize. Shortly afterwards, Enrico Fermi discovered that irradiating elements with neutrons transformed them into other elements. The Nobel Prize was also awarded to Otto Hahn for his discovery (1938), which, building on the existence of neutrons and Fermi's discovery, showed that neutrons could be used to split nuclei with high mass. Very shortly afterwards, the first controlled chain reaction was created under the leadership of Enrico Fermi, with the help of Leo Szilárd and others. Today, after a few decades, about 50% of the electricity produced in Hungary comes from chain reactions. Chain reactions, and the neutrons they produce, are used for research, education and energy purposes all over the world.
During the session, we will first visit the BME's Teaching Reactor, where we will learn about different experimental techniques related to the reactor. Afterwards, sitting in front of a simulator showing the behaviour of the primary circuit of the Paks nuclear power plant, everyone will have the opportunity to control the plant: to virtually reduce or increase the power, or even to cause a malfunction. We will try to understand what it takes to make a nuclear power plant work safely.
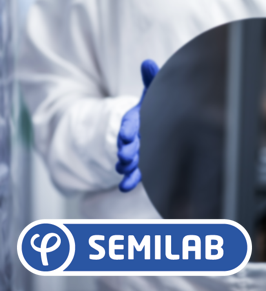
Semiconductor Technology From the First Transistor to the Smartphone
Inventions from the semiconductor industry are all around us in everyday life, from computers to mobile phones, cars or even a simple calculator. They all contain transistors, for example, the basic building blocks of modern electronics. John Bardeen, Walter Brattain, and William Shockley won the Nobel Prize in 1956 for the development of transistors. Another Nobel Prize-winning semiconductor invention (2009 - William S. Boyle, George E. Smith) is the CCD image sensor, which has been a breakthrough in digital imaging and is still an essential component of modern cameras.
At Semilab, one of the largest development companies in Hungary, we manufacture measuring equipment for the semiconductor industry. At our company, we come across these Nobel Prize-winning semiconductor devices every day, either through our contacts with their manufacturers or by incorporating them into our own equipment. A visit to us will give you a better understanding of image sensors and their modern applications. You will see machines that use these sensors to image the surface of semiconductor samples and their defects for quality inspection, and we will also show you how they contribute to the development of imaging sensors, which is still actively underway.